By definition, a mineral is a natural solid, generally formed by inorganic processes, with an ordered arrangement of elements and with a fixed chemical composition and physical properties that vary over a limited range. In nature, there are several geological contexts where we generally find different minerals forming three types of rocks: sedimentary, metamorphic and igneous. In sedimentary environments (coasts, rivers and lakes, among others), those in which sedimentary processes develop in contact with the Biosphere, we can find beautiful exceptions to the original definition of mineral in relation to its formation. In general, microorganisms (autotrophic eukaryotes and prokaryotes, autotrophs and heterotrophs) in lake systems form laminated benthic microbial communities called microbial mats. These mats trap and stick detrital grains (from the weathering and erosion of surrounding rocks) or induce the precipitation of authigenic minerals, that is, those that precipitate in situ. Precisely, in microbial mats and other living beings, it results in the bioinduced or biocontrolled precipitation of minerals. The most common biominerals are carbonates (aragonite, calcite and dolomite), although phosphates (apatite), clay minerals (smectites and palygorskite), some oxides (magnetite), sulfides (pyrite) and sulfates (gypsum and hexahydrite) can be equally bioinduced or biocontrolled.
What is the difference between bioinduced and biocontrolled mineral precipitation?
According toDupraz et al. (2009), these two types of biomineralization are differentiated based on the prevalence between two types of factors: intrinsic or related to microbial or cellular metabolism (Ex: photosynthesis, respiration and bone formation) and extrinsic or related to the environment (Ex: desiccation and degradation). If the first factors predominate over the second, we are talking about controlled biomineralization, that is, the metabolism of bacteria, some autotrophic eukaryotes (such as diatoms) and heterotrophs is the promoter of mineral nucleation and subsequent crystalline growth. For the general public, some examples of clear biominerals close to our experience and the result of metabolic control are: the shells of invertebrates such as gastropods, composed of calcium carbonate (aragonite or calcite), the frustules of diatoms (composed of opal) or the bones of vertebrates, composed of calcium phosphate (apatite). It is clear that these biominerals have a physiological function for the organism that produces them (protective or structural), which regulates the growth or dissolution of these crystals (Ex: our bones).
Many other extracellular mineral precipitates, in fact, can be considered products of bioinduced mineralization. What is the difference with the previous ones?
Extracellular polymeric substances (EPS) are the result of interactions between microorganisms and their environment.(Decho, 1990).EPS are produced in great abundance in microbial mats developed in response to extreme conditions of salinity, pH and temperature and which control their production.(Dupraz and Visscher, 2005).If, based on the chemical elements linked to the functional groups of the cell walls or in the extracellular polymeric substances, certain extrinsic processes, such as the desiccation or degradation of other microorganisms, are the triggers of mineral nucleation, then we will be analyzing bioinduced minerals. . This is the case of many minerals in a lake environment (carbonates, sulfates and some clay minerals), where evaporation induces crystallization within these organic substances in contact with the brine.(Cabestrero and Sanz-Montero, 2018; del Buey et al., 2018, 2021).
Due to the complexity of establishing a clear boundary between intrinsic or extrinsic factors in terms of extracellular precipitation, we designed microbial cultures to limit the variables involved in sedimentary environments. These microbial cultures can be from an entire microbial community or from an isolated genus or species. The latter are intended to evaluate a certain metabolism in mineral precipitation (oxygenic, anoxygenic photosynthesis, sulfate-reduction). Knowing the composition of the culture medium and having controlled the variables of light/dark, temperature and salinity, we can precisely determine the biomineralization mechanism for a certain mineral and a species of bacteria.(Sánchez-Román et al., 2007, 2008, 2011).
How do we study the textural relationships between minerals and microorganisms? How can we identify microorganisms?
Geobiology, when studying samples with a variable proportion of biological tissues with different degrees of mineralization, requires sample preparation more related to histology or microbiology than to mineralogy. On the contrary, X-ray diffraction continues to be the basic technique for mineralogical determination in this type of samples, as minerals are the ultimate research objective. No less important is scanning electron microscopy (SEM), which allows us to recognize textural and chemical features between cell walls and minerals. For a study of more detail and resolution, transmission electron microscopy (TEM) allows studying textural relationships at the nm scale. The preparation of this type of samples, both natural and cultured, for electron microscopy requires a prior fixation (glutaraldehyde) and dehydration (ethanol) procedure. In the case of transmission electron microscopy, as electrons pass through the sample, cut by ultramicrotome into ultrathin sections, stains with heavy elements (Os, Pb and U) are used, which allow the contrast between the organic matter (solid and visible) and mineral (opaque).
In parallel, identifying the taxonomy of the groups that make up a microbial tapestry from the field or one from a culture medium requires analyzes based on the gene that codes for the 16S ribosomal RNA. Using operational taxonomic units (OTUs), sequences with a homology of more than 97% are grouped and compared with specific databases for microbial mats. Alternatively, FISH (Fluorescence In Situ Hybridization) can be used, which allows, through a set of fluorescence-active oligonucleotide sequences that bind to the rRNA of living cells, to identify the bacterial phyla present in microbial mats.(Cabestrero et al., 2018).
And all this, why do we study it?
In Geology we apply the Principle of Actualism (sorry, we don’t have our own laws…). What does it tell us? That all the geological processes that we see today have also occurred in the Earth’s past. That allows us? Being able to interpret the geological record (rocks) based on the processes we see today. Furthermore, if we can study the interactions between living beings and the Geosphere in the present, the more accurate our inferences will be from the biotic markers preserved in the rocks. Where can we go? We can go back in the geological past of the Earth to the oldest manifestations of microbial life: stromatolites (2,700 Ma), laminated and lithified microbial communities. And further into space? The study of biominerals can even transcend the scope of other planets, such as Mars, where the association of clay minerals with carbonates(Morris et al., 2010)and sulfates(Ehlmann et al., 2011)It has been detected through spectral analysis in some regions of the red planet.
References:
Cabestrero, Ó. and Sanz-Montero, ME, 2018. Brine evolution in two inland evaporative environments: influence of microbial mats in mineral precipitation. J. Paleolimnol. 59 (2), 139-157.
Cabestrero, Ó., Sanz-Montero, ME, Arregui, L., Serrano, S., Visscher, PT, 2018. Seasonal variability of mineral formation in microbial mats subjected to drying and wetting cycles in alkaline and hypersaline sedimentary environments. Aquat. Geochem. 24 (1), 79-105.
Decho, A.W., 1990. Microbial exopolymeric secretions in oceans environments: their role(s) in food webs and marine processes. Annu. Rev. Oceanogr. Mar. Biol. 28, 73-154.
Del Buey, P., Cabestrero, Ó., Arroyo, X., Sanz-Montero, ME, 2018. Microbially induced palygroskite-sepiolite authigenesis in modern hypersaline lakes (Central Spain). Clay App. Sci. 160, 9-21.
Del Buey, P., Sanz-Montero, ME, Braissant, O., Cabestrero, Ó. Visscher, PT, 2021. The role of microbial extracellular polymeric substances on formation of sulfate minerals and fibrous Mg-clays. Chem. Geol. 581, 120403.
Dupraz, C. and Visscher, P.T., 2005. Microbial lithification in marine stromatolites and hypersaline mats. Trends. Microbiol. 13, 429-438.
Dupraz, C., Reid, R.P., Braissant, O., Decho, AW, Norman, R.S., Vissher, P.T., 2009. Processes of carbonate precipitation in modern microbial mats. Earth Sci. Rev. 96, 141-162.
Ehlmann, JF, Mustard, JF, Murchie, SL, Bibring, JP, Meunier, A., Fraeman, AA, Langevin, Yves., 2011. Subsurface water and clay mineral formation during the early history of Mars. Nature, 479, 53-60.
Morris RV, Ruff S., Gellert R., Ming DW, Arvidson RE, Clark BC, Golden DC, Siebach K., Klingelhöfer G., Scröder C., Fleischer I., Yen AS, Squyres SW, 2010. Identification of carbonate -rich outcrops on Mars by the Spirit rober. Science, 329, 421-424.
Sánchez-Román, M., Rivadeneyra, M.Á., Vasconcelos, C. and Mckenzie, JA, 2007. Biomineralization of carbonate and phosphate by moderately halophilic bacteria. FEMS Microbiol. Ecol. 61, 273-284.
Sánchez-Román, M., Vasconcelos, C., Schmid, T., Dittrich, M. and McKenxie, JA, 2008. Aerobic microbial dolomite at the nanometer scale: implication for the geological record. Geology 36, 879-882.
Sánchez-Román, M., Romanek, CS, Fernández-Remolar, DC, Sánchez-Navas, A., McKenzie, JA, Amils Pibernat, R. and Vasconcelos, C., 2011. Aerobic biomineralization of Mg-rich carbohydrates: implications for natural environments. Chem. Geol. 281, 143-150.
Can you help us to become more? Become a member and participate. Spread our word on the networks. Contact us and tell us about yourself and your project.
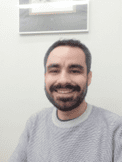
Pablo del Buey Fernández
Postdoctoral Researcher at Vrije Universiteit Amsterdam
Nací y crecí en Madrid, donde estudié en la Universidad Complutense el Grado en Geología. Institución en la que también he cursado todos mis estudios de postgrado hasta doctorarme en 2022 en el Departamento de Mineralogía y Petrología. Desde que estaba cursando el Grado en Geología me vinculé con la investigación en mineralogía, primero en crecimiento cristalino y durante la Tesis Doctoral en biomineralización en tapices microbianos. A lo largo de mi etapa predoctoral he tenido la oportunidad de realizar dos estancias breves en el extranjero, la primera en 2017, en el Department of Biomedical Engineering (University of Basel), donde aprendí a caracterizar fisicoquímicamente las sustancias poliméricas extracelulares (en sus siglas en inglés, EPS). Posteriormente, en 2019, realicé otra estancia predoctoral en el Department of Earth Sciences (Vrije Universiteit Ámsterdam) trabajando con cultivos microbianos fotosintéticos. En esta última institución, durante el 2023, estoy realizando mi primera estancia postdoctoral como becario Margarita Salas-UCM. En la actualidad, estoy desarrollando con mayor énfasis una de las líneas de investigación que abordé en la Tesis Doctoral, la mineralogía de arcillas de sistemas lacustres, trabajado en coordinación con el Departamento de Mineralogía y Petrología de la Universidad de Granada.